Before multidisciplinary approaches in the study of water had become popular, Professor Greg Characklis was thinking about how to better integrate environmental science, engineering, and economics in his research. After an initial (short) stint in the private sector (at Azurix Corporation), in 2001 Greg Characklis joined the faculty of the University of North Carolina in Chapel Hill, where he has been ever since. A prolific scholar, Characklis has argued that risk is a key factor that connects environmental, engineering, and economic questions, and he has worked especially on ways to mitigate water-related risks. Greg Characklis leads the Center on Financial Risk in Environmental Systems at UNC. He gave a talk at the University of Virginia about “Managing Environmental Financial Risks through Modeling of Coupled Natural Human Systems,” in which he focused in particular on the drought risks that water utilities face. We spoke with him:
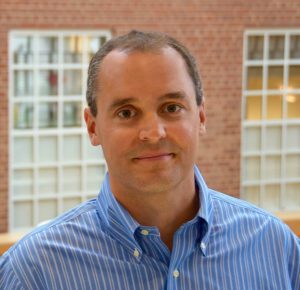
Philip C. Singer Distinguished Professor
Department of Environmental Sciences
and Engineering
Director
Center on Financial Risk
in Environmental Systems
Why do water utilities care about droughts, and what is their attitude toward risk?
At the heart of the question is a mismatch between the water utilities’ structure of costs (mostly fixed) and revenue (mainly volumetric, variable revenue). In addition, water utilities tend to be very risk averse both because of their crucial role in securing public health and because, as non-profit maximizing, public sector entities, they have little incentive to take risk.
Water utilities have two primary goals. They protect public health by providing safe water, and they deliver that water with a high level of reliability. To ensure high reliability, most have historically maintained enough supply capacity to meet demand under even the most extreme droughts. This results in a significant amount of capacity that is infrequently used, yet still incurs costs and imposes environmental impacts, particularly in the case of surface water (which serves the majority of Americans), which are typically managed by reservoir/dam systems.
Building additional reservoir capacity has become increasingly expensive in recent decades, because of the declining number of attractive sites and the increasingly stringent environmental regulatory approval process. Consequently, communities seek to manage their drought-related supply risks through conservation (both temporary and long-term) and by acquiring water from others during periods of scarcity. This typically involves transfers of water. In the western United States, agriculture tends to supply the transferred water, whereas in the east, utilities may get transfers from neighboring utilities, with the volume traded varying based on differing supply-to-demand ratios and the proximity of neighboring systems.
While conservation and reallocation of existing water resources could be viewed as better ways of managing drought, they have one significant drawback: they impose more financial instability. Conservation reduces the utility’s water sales, while transfers increase costs. Moreover, as these are responses to intermittent drought, they come at unpredictable intervals. Note that such fluctuations can be large and difficult to manage, especially because of the cost-revenue mismatch. Most of of a utility’s costs (often 50% or more) are tied to debt-service payments and other fixed costs that do not vary much even if water sales, which generate most of their revenues, decline. A utility’s standard financial model also revolves around cost recovery, such that prices are set at a level that ensure its costs (mostly predictable) are covered. In addition, for a public-sector utility, there are challenges associated with rapidly increasing prices to compensate for lower revenues and/or higher costs during drought. Because of all these factors, utilities are searching for new management strategies that integrate consideration of both water supply risk and financial risk.
What could be the role of index insurance in order to mitigate the risks water utilities face? And does this opportunity to hedge water risk currently exist?
Utilities have a number of tools to manage drought-related financial risk.
Drought-pricing schemes can be instituted well in advance of a drought to compensate for the lower revenues or increased costs of droughts. As noted, public utilities are reluctant to rely too much on them to promote financial stability. This is likely because of the public-sector nature of water utilities, the adverse customer response, and concerns over the impact on low-income households.
Utilities with block-rate pricing will sometimes place large surcharges on high-volume users (and little to none on low-volume users). Because of the price sensitivity (higher price elasticity) of the large block users, they sometimes find—to their surprise—that this has little impact on or even reduces revenues.
Another option to achieve greater financial stability is to increase the fraction of fixed versus volumetric charges in water pricing. However, this alternative has been discouraged by regulators in many regions, such as California, as it erodes incentives to conserve water.
Of course, all utilities maintain cash reserves as a buffer against uncertainty. While some have dedicated “revenue stabilization” funds, most compensate for budget shortfalls by drawing from general funds intended for maintenance and capital projects, sources that are already underfunded in most cases. Regardless, maintaining funds large enough to protect against more extreme and/or multiyear droughts can have very high opportunity costs given the need for liquidity and the commensurately low returns. It is in this context that the discussion has recently turned to the potential for financial instruments, such as index insurance, that can play a role in managing the financial risks of drought, particularly in the case of more extreme droughts. While an indemnity-based instrument (e.g., auto collision insurance) could work in these situations, it is subject to substantial moral hazard as it could induce more risky behavior by the utilities.
Therefore, interest has increased in index-based instruments. These could be cheaper, presuming the identification of a transparent index, and thus an instrument that cannot be influenced by the utilities, reducing or eliminating moral hazard concerns. The effectiveness of these instruments is, however, highly dependent on identifying a measure (index) that is well correlated with utility revenues and/or costs, and this is not trivial. A relatively simple index, such as rainfall, does not typically correlate well with utility financial metrics. Hence, a financial instrument built around such an index would be subject to high levels of “basis risk,” meaning that the buyer would receive payouts that would not match up well with his/her losses. My group at UNC spends a lot of time trying to develop effective indices, because this both requires a good understanding of the sources of financial risk and provides a strong basis for developing a financial instrument.
Our overall objective is to develop integrated drought-management strategies that might include conservation, acquiring new supplies (i.e., transfers), drought pricing, a reserve fund, and a financial instrument. All these options combined should help meet specified levels of supply reliability and financial stability at an acceptable cost. In the work my group has done with utilities, we have found that financial instruments can be an important part of an integrated strategy, particularly when used to protect against the financial consequences of low-frequency, high-consequence events, such as extreme and/or multiyear droughts. What we are developing is not unlike the role that financial instruments have played in the electric-power sector, such as contracts linked to “Heating Degree Days” and “Cooling Degree Days,” indices that are used to measure temperature deviations from historic norms. Such deviations are known to correlate well with electricity demand and utility revenues. It is worth noting, however, that electric-power utilities experience somewhat different circumstances than water utilities do, in that electric-power utilities often have significant control over supply, even as they are subject to variability in demand. Water utilities experience variability in both supply and demand (or perhaps it would be more accurate to say water consumption here), and these are often negatively correlated, making the financial consequences of drought more difficult to manage in many ways.
You have done very innovative work in North Carolina’s research triangle (some of it with our UVA colleague Larry Band). How does the North Carolina case fit in here? And what is the role of water transfers?
Like many regions in the eastern part of the United States, there are a number of population centers, and thus water utilities, that operate in close proximity. Each utility is typically independent and maintains its own water-supply system. Importantly, the supply-to-demand ratio tends to vary by utility, as each utility has expansions to its water supply at different times, and reservoirs are built to meet demand several decades in the future. What we figured out is that even during a drought, there are often one or more utilities in a region with sufficient water supply to transfer some of their surplus to neighboring systems, and in most cases, to receive some compensation for this service.
The ability to transfer water depends on having the appropriate conveyance infrastructure in place, which is more likely the closer the utility is to its neighbor. Such a reallocation of existing supplies during a drought can be a valuable tool in alleviating drought conditions. The costs of these intermittent transfers, however, is not often a part of a utility’s budgeting process, and thus imposes financial risk on the system. In addition, the water-scarce utility (the one buying the transfer) is usually already imposing conservation measures on its customers and thus seeing a reduction in revenues as well. Nonetheless, there can be a trade-off here, in that the transferred water that is sold to the purchasing utility’s customers may compensate for some portion of the revenue lost via conservation. In conclusion, the reallocation of water resources during drought via transfers (whether originating from nearby utilities in the eastern states or agricultural irrigators in the western states) can be a valuable tool. It can help manage the drought-related supply risk. At the same time, this approach also imposes financial risk that must be managed if utility managers are going to use this tool to its fullest capability, which is where the use of index insurance comes in.
You have been on record arguing in favor of the integration of disciplines. In particular, you once co-authored an article called “Increasing the Role of Economics in Environmental Research (or Moving beyond the Mindset that Economics = Accounting).” What point did you want to make by writing this article?
Decisions about society’s most challenging environmental problems hinge upon both technical and economic arguments. Environmental engineering students have the potential to contribute a great deal of expertise to solving these problems, but they would be much more effective if they had an improved understanding of concepts in both disciplines. At the time the article you refer to was written (2011), too much of that potential was unrealized. And, while things have improved a bit, the environmental engineering community still has a long way to go in terms of preparing its students to fully pursue interdisciplinary opportunities aggressively.
One of the challenges, as alluded to in the title of the article, is that engineering students often have little idea of what the field of economics has to offer. They often view it as a form of accounting instead of the study of the institutions that allocate scarce resources (an economist’s definition). This is partly a consequence of packed engineering curricula, but is also a byproduct of a required and useful, but poorly labeled, course present in all of these curricula, Engineering Economics. In most cases, this course has little to do with economic theory, institutions, or the pursuit of efficiency, but instead focuses on topics of practical use to engineers such as project finance and discounted cash flows. (By the way, I’m not knocking the content of this course, only its misleading title).
As a result, many engineering students leave this course believing that they know what “economics” is all about, which in some cases is worse than if they were to remain entirely ignorant with respect to the field. As a result, it takes me half a semester to deprogram my engineering students in my water resource systems course so that they can begin their understanding of economics afresh. Once I do, I find that the logical underpinnings and mathematical orientation of economics are very appealing to many of them. These features allow engineering students to rapidly operationalize economic concepts, and many are quickly in a position, with some help, to begin identifying opportunities for integrating these concepts into their engineering research in a manner that yields interesting insights.
For those interested in exploring this further, I find the investment of time required to reach a productive level of knowledge is relatively modest. A single course in microeconomics, followed by a course in natural resource/environmental economics, is usually enough to put engineering students in a position to recognize and/or formulate interesting interdisciplinary research questions. At that point, they can seek out collaborators in economics and begin a productive inquiry. This investment of time may not be right for all engineering students, but my experience has been that a significant number of them, and many of the most talented, find this type of interdisciplinary research to be exciting. They are often more than willing to put in the time.
Given the nature of undergraduate engineering curricula, it may make the most sense for a short sequence in economics to wait until the graduate level. However, making these courses readily available to interested students would likely yield a new breed of researcher. It may also serve to revitalize some traditional programs that have grown stale. Given the rapid increase in funding for interdisciplinary water research over the last 5+ years, such a curricular change could yield significant benefits in the research realm as well. In a period in which many academic departments are seeing shrinking budgets, an influx of talented students and heretofore untapped research funds could provide ample incentive for early adopters.