Electrochemistry and Water
There are three main processes to consider when discussing clean water. The first is acquiring clean water for consumption. Hydrology is a deciding factor regarding the availability of water from sources like lakes, rivers, aquifers, or oceans. Chemistry and electrochemistry are used in the process of refining and treating water to a level safe for either human or industrial consumption. One example of electrochemistry’s impact on clean water availability is electrolytic desalination, which is the process of purifying saline water in an electrochemical cell.
The second process is delivering clean water as responsibly and efficiently as possible. This includes policy decisions surrounding water distribution, water use, and extenuating stressors like natural disasters (either acute, like tsunamis and earthquakes, or chronic, like droughts, pandemics, and aging infrastructure).
The third important process involves maintaining a desirable level of water cleanliness, which includes protecting natural deposits of fresh (near-clean) water from contamination and preserving water that has already been treated and is ready for consumption. Corrosion can cause degradation of structures designed to contain oil and other fuels, hazardous wastes, chemicals, and so on, which can be released and then go on to contaminate natural waters. “Clean” (treated) water is commonly compromised due to the growth of biological contaminants or the release of hazardous chemicals from structural materials like plastics, metals, and ceramics. Disinfectants (chlorine or chloramines) can enhance corrosion. Metallic corrosion is particularly important for systems containing metallic structures (pipes, valves, solder, etc.) and corrosive water chemistries. One unfortunate example of this is the Flint water crisis.
Flint’s Water Crisis
Extensive iron and lead corrosion of the water distribution system in Flint, Michigan, created “red water” complaints, rapid loss of sanitation capacity, and an outbreak of Legionnaires’ Disease that killed 12 people. These acute symptoms masked the underlying problem of lead release in the water, which went undiagnosed for about a year. The repercussions have been tragic. In all, state and federal agencies have disbursed $450 million in aid so far. In August, the state of Michigan reached a mediated settlement in a civil suit and is expected to pay about $600 million to victims, many of whom are children facing potential long-term consequences from lead exposure.
The Flint story is a cautionary tale of too little too late, and of reactionary actions not driven by scientific data. While there are many reasons for the disaster, we will highlight the increased salinity and acidity of the water (i.e., water corrosiveness) and the suspended use of corrosion inhibitors. The whole crisis began with the decision to switch city water providers in order to save money. While the logistics of the switch were being worked out, the city reinstated its own outmoded water plant to treat water from the Flint River for its short-term needs. Needless to say, the facilities and the personnel were not up to the task. The data shows that the acidity of the drinking water—meaning the treated water, not the river water—steadily increased (its pH decreased) and its chloride-to-sulfate mass ratio (CSMR) steadily increased. This increased acidity and salinity (specifically regarding chloride) was a major cause of the crisis because it increased lead corrosion, as discussed below.
Water’s acidity (pH) is crucially important in determining corrosion rates, as it affects the stability of corrosion-limiting films on the surface of the metal pipes. Chloride is another “bad actor” that can also destabilize the partially protective films and facilitate excessive localized corrosion. The corrosion rate in drinking water systems is largely limited by the conductivity of the drinking water (which is normally low). Neither the acidity nor the CSMR of the treated water was flagged in the months leading up to the watershed lead release and final exposé of the lead-in-water levels.
Additionally, Flint had made the policy decision not to use phosphate-based corrosion inhibitors in order to save costs. Phosphate treatment is intended to limit lead release through the formation on the pipe walls of an insoluble lead phosphate film with a low lead level. While there are pros and cons of adding foreign chemicals to drinking water (fluoride, for example, comes to mind), the efficacy of phosphate as a corrosion inhibitor for lead is well known and not really questioned. In Flint, the cost savings of not using the inhibitor (~$100/day) were not justified given the resulting human health crisis. In addition to the incalculable damage done to the quality of human life, monetary costs alone highlight the folly of this decision (compare $100/day to $1 billion; see above). A consultation of either the literature or chemical diagrams could have helped clarify why phosphate is so important.
Chemical Thermodynamics Can Explain
Chemical thermodynamics can predict the formation of stable lead-based compounds on lead pipe surfaces. Certain compounds form advantageous films that might act as kinetic barriers to hinder corrosion and function to sequester otherwise soluble lead. Stable film development depends on a certain equilibrium chemistry that is relevant for phosphate treatment. Add more phosphate, and you can remove more soluble lead to form a protective lead phosphate film. Remove phosphate completely, and you have to hope that other lead compounds (lead-carbonate, -sulfate, -oxide, etc.) can remove the levels of lead you need. However, these other components cannot achieve an equilibrium lead concentration below the EPA Action Level of 15 parts per billion (dashed horizontal line in the thermodynamic diagram below). Only the phosphate compounds can establish equilibrium lead concentrations below the EPA’s Lead Action Level. But it takes time even for the best scale to form. Phosphate anions can inhibit corrosion rates but this can only delay lead accumulation. Corrosion engineers need to explore new inhibitor chemicals and surface-altering agents that can optimize the protective scale coverage on a pipe wall.
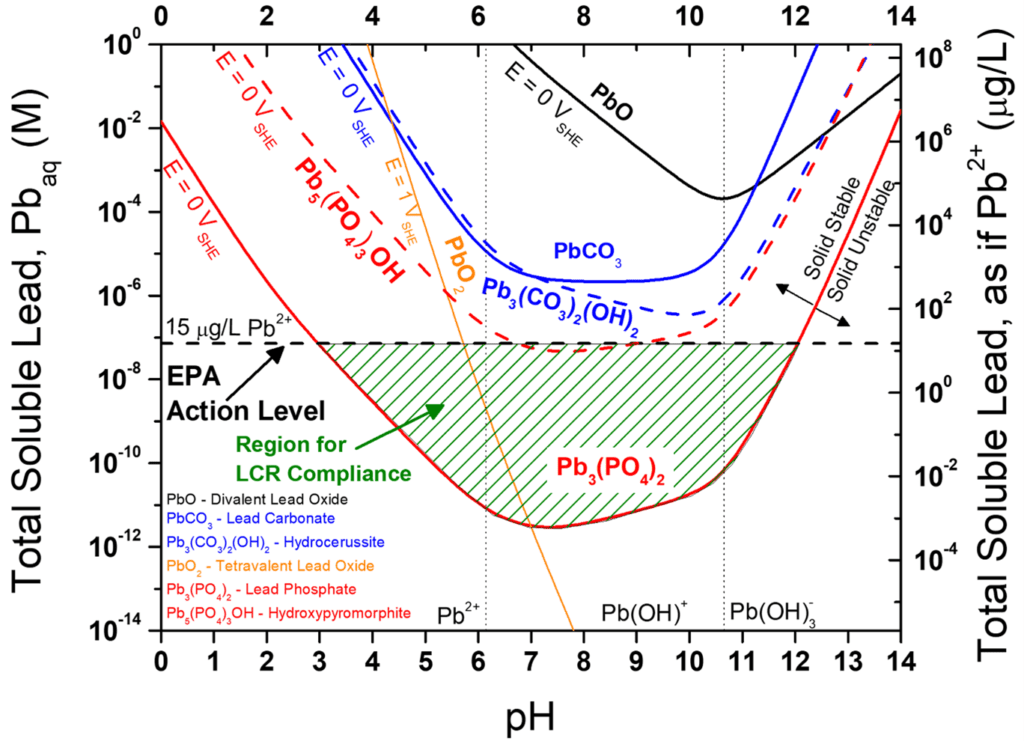
Bigger than Flint, Our Common Threat
Lead poisoning from degrading lead pipes is a pervasive threat, as lead piping was used extensively in the United States. Future incidents are likely.
Some argue that the investment in scientific research and modeling is unnecessary because lead-based pipes are being replaced, albeit at homeowners’ expense. While the total replacement of lead service lines is a wonderful goal, finding all sources of lead can be difficult. Replacing lead in public water systems does not simply mean replacing lead pipes. Additional sources of lead include lead-based solder used to join pipes together, commercial brass, which commonly contains small amounts of lead, and lead ions that soak into the corrosion film of steel pipes over time. Furthermore, partial replacement of lead pipes with steel or copper can actually increase lead release due to galvanic corrosion, a process where contact between two dissimilar metals causes protection of one and accelerated degradation of the other (like the protection of steel by zinc in galvanized systems but in reverse, with lead being corroded by copper or steel).
A Way Forward
We need a fresh perspective that focuses on what lies around the curve ahead. Commonly proposed strategies offer false comfort based on sparse testing, rules of thumb, and conventional wisdom, rather than on sound scientific principles. Risk assessments based on scientific data should replace the current reliance on periodic water sampling, which is imprecise and often too late to prevent a disaster. Current infrequent sampling cannot catch an exposure as it happens. It cannot predict, only diagnose.
Managers and regulators of public water systems should be educated on corrosion fundamentals and have tools at their disposal to predict the risks of lead release. A predictive framework for lead corrosion would allow regulators and managers to anticipate problems and manage the risk of water conditions associated with unacceptable lead release. Such frameworks could generate risk assessments based on dynamic data about water chemistry, reaction rates, scale formation, and inhibitor corrosion mechanisms, as well as water stagnation and flow. Current skeletal frameworks exist to guide water treatment decisions based on limited criteria like water pH and dissolved inorganic carbon (see the example from the EPA cited below). These decisions are likely based on many years of anecdotal experience and probably yield good results most of the time.
Ultimately, holistic, kinetic models that incorporate the rate of lead release from all possible sources, under many real-world operating conditions, are needed. The root cause of the Flint water crisis can be found at the intersection of materials science, surface science, water chemistry, and electrochemistry. We need to better understand the scientific factors that govern lead release, and that starts with a better testing strategy and more complete understanding of some fundamental truths. A better-informed society can prevent such disasters from happening in the future through improved risk assessment, including especially the anticipation and management of factors affecting lead release. We all have a part to play in averting future lead poisoning disasters.
John Scully is a Professor of Engineering at the University of Virginia and the co-Director of the Center for Electrochemical Science and Engineering. Raymond Santucci is an Assistant Professor at the Naval Academy.
For those who are interested in learning more about the Flint water crisis and the pervasive threat of lead in drinking water, please consult:
- John R. Scully, “The Corrosion Crisis in Flint, Michigan: A Call for Improvements in Technology,” in “Issues at the Technology/Policy Interface,” ed. Ronald M. Latanision, special issue, The Bridge 46, no. 2 (July 1, 2016), https://www.nae.edu/155382/The-Corrosion-Crisis-in-Flint-Michigan-A-Call-for-Improvements-in-Technology- (accessed Sept. 20, 2021).
- CORROSION Assigns “Editor’s Choice” Open Access to Key Papers Related to the Water Crisis in Flint, Michigan, J.R. Scully, Corrosion Journal, National Association of Corrosion Engineers, 2016
- Raymond J. Santucci Jr. and John R. Scully, “The Pervasive Threat of Lead (Pb) in Drinking Water: Unmasking and Pursuing Scientific Factors that Govern Lead Release,” Proceedings of the National Academy of Sciences 117, no. 38 (September 2020), https://www.pnas.org/content/117/38/23211 (accessed Sept. 20, 2021).
- “Correction for Santucci Jr. and Scully, ‘The Pervasive Threat of Lead (Pb) in Drinking Water: Unmasking and Pursuing Scientific Factors that Govern Lead Release,’” Proceedings of the National Academy of Sciences 118, no. 34 (August 2021), https://www.pnas.org/content/118/34/e2112469118 (accessed Sept. 20, 2021).
- “Optimum Corrosion Control Treatment Evaluations Technical Recommendations for Primary Agencies and Public Water Systems,” Environmental Protection Agency EPA 816-B-16-003